Wrapping up the latest work on the Organised Atoms project, here are some curve traces of natural semiconductors found in the wild amongst the tailings dumps of some of the many thousands of abandoned mines in Cornwall. These experiments were carried out as part of a study into the basis underlying the more playful activities of our workshops with young families at United Downs Raceway, listening to crystals by incorporating them into simple synthesiser circuits.
It was important for us to spend some time doing this to provide a stable scientific understanding - as the area of crystals is rife with pseudo-science, and we wanted to be able to explain what we were doing in the basis of early radio experiments with semiconductors with confidence.
These plots were measured by passing a voltage between -2.5 and +2.5 V (a rising saw tooth wave at 1Khz) across the crystal material via a stainless steel 'cat's whisker' tickling the surface of the crystal providing a point contact, with positive going to the cat's whisker and negative going to the crystal substance. The corresponding voltage drop across a series connected 1K resistor to ground was measured to calculate the current. We used a Multicomp Pro Digital Storage 2 Channel Oscilloscope (MP720783) to measure and store the data.
Firstly here are a few comparison plots of factory made electronic components.
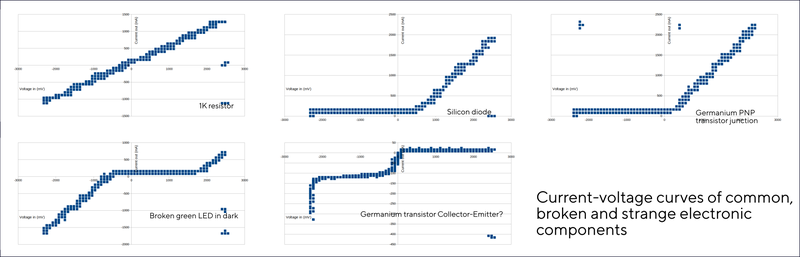
Resistors are not semiconductors, and so have show straight lines. As the resistance increases the flatter the line is (perfect insulators would be a horizontal line, while a perfect conductor would be a vertical line). Semiconductors provide non-linear response, so are 'bendy' instead of straight - silicon and germanium diodes are flat (behave like insulators) when the voltage is negative, but behave like resistors when they are positive, above a certain threshold. Germanium starts conducting at a slightly lower voltage than silicon, as can be seen here.
We've also plotted some strange behaviour with a broken LED - which when wired up backwards (and in darkness!) starts conducting at around 2V in addition to its normal behaviour. The last plot shows a very small current leaking across a (very old and British made) germanium transistor junction in a strange way.
Now lets look at some naturally grown semiconductors - these are all crystals grown during the "variscan orogeny" in the late paleozoic era, 270 - 350 million years ago. They were mined from hundreds of metres below the surface, existing for most of that time in dry conditions below the water table, and were brought up and discarded near to the mine shafts, as they were worthless to the miners.
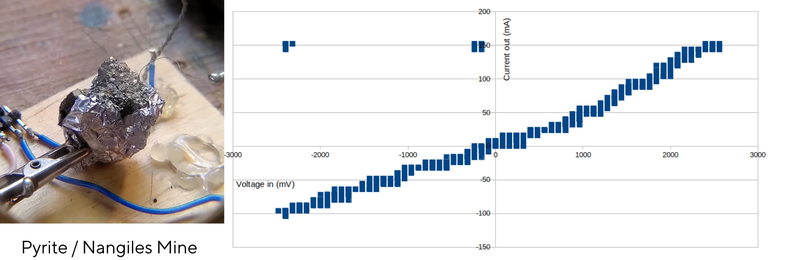
This is a piece of well degraded pyrite from Nangiles mine, which was actually worked up to fairly recently - it only closed in 1991. Here it shows a very slight upward curve in the right hand, positive half of the graph. At -2.5 V it allows 100mA to pass through it, while at +2.5V it's reading 150mA - so it is semiconducting, but not nearly as well as the factory made components.
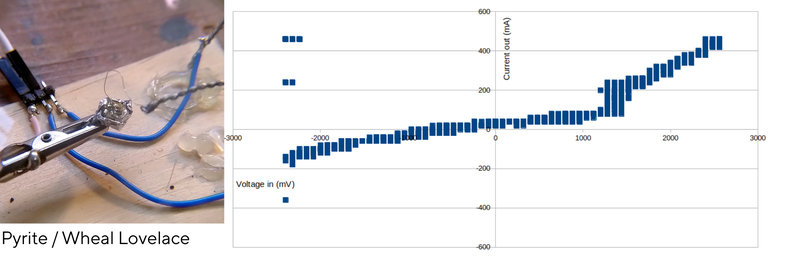
This is the same mineral, pyrite - but from a different, older mine - Wheal Lovelace. Here the material on the surface is likely to be from around 1780 or before, and despite having been exposed to water for longer, seems to have a more intact, courser crystalline formation than Nangiles above (despite it being only a few miles away). We see much less conductivity in the negative section, which extends quite a way into the positive half, until we reach 1.5V when the current suddenly jumps up to 500mA at +2.5V.
We've found pyrite in general to provide a range of different behaviours and seems to get more semiconducting if left to build up a surface oxidisation, while clean breaks seem less useable.
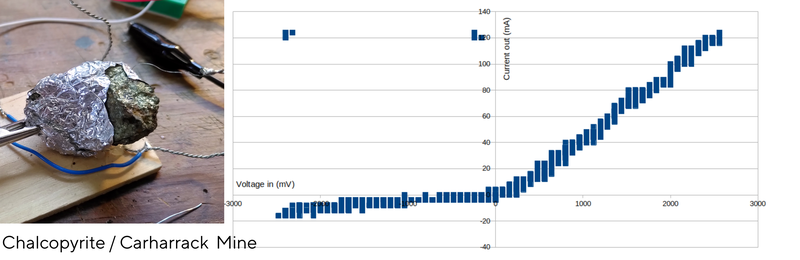
This specimen of chalcopyrite (copper ore) from Carharrack Mine is providing a very similar behaviour in curve shape to our factory made silicon or germanium diodes. In fact it appears to start conducting at an even lower voltage than germanium, but it's difficult to tell with any precision with our methods. The current it allows through is lower than the pyrite above however, as it's only passing 120mA at +2.5V.
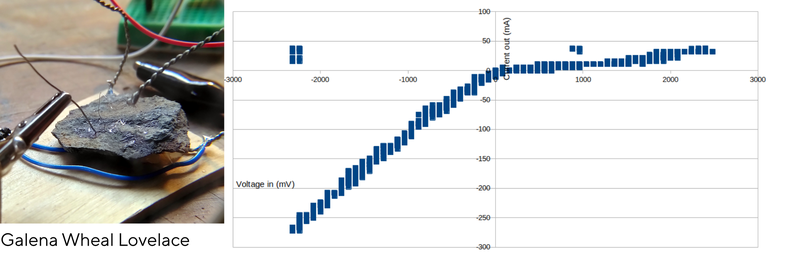
Also from Wheal Lovelace, this is galena (lead ore), a mineral which was traditionally used in radio receivers in the early 20th century. The graph is upside down as I've kept them consistent in regard to how they were wired up, but some minerals behave differently in polarity - apparently due to their impurities. In terms of oxidisation, galena seems the reverse of pyrite, in that freshly broken crystals work well, but degrade over time if exposed to air and humidity.

Some relatively rarer minerals next, this one is a wolframite mineral (probably ferberite) which is an ore of tungsten. This specimen is from Poldice mine (an important copper and tin mine) and semiconducts very well, allowing up to 1A to flow at +2.5V and low negative leakage in comparison. In the past we've seen wolframite show 'negative resistance' where it decreases in current as the voltage increases, which is an unusual property used in tunnel diodes. We couldn't replicate that here, but it did show some very noisy characteristics with a symmetrical curve on the right. This is an example of how the same crystal can show very different behaviours depending on where it touches the cat's whisker wire - it can take some time to find good locations, and there can be almost as much variability on a single piece of material as between the minerals themselves.

Chalcocite from Penstruthal mine shows some of the most impressive semiconducting properties we've found. Here again two point contact locations on this crystal are graphed. The interesting thing with chalcocite is that it takes quite a high voltage to make it conduct, when it jumps very quickly to high current levels - but once 'turned on' in the positive region, it will carry on conducting until the voltage can drops all the way back to zero, when it 'turns off' again. Here it is allowing 1.6A to flow at +2.5V.
Chalcocite's properties became apparent initially by listening to it, as it was obvious while playing with different minerals while designing audio circuits for the workshop that it was different to other minerals. This is a recording made by passing signals across the same crystal being tested above. These recordings are more like field recordings than music being 'played', as you can set them up, let them run and record anything interesting happening. Most of the textural changes are due to unknown instabilities in the naturally grown crystal and the point contact connection: temperature, light and atomic fluctuations interacting with the already unstable CMOS circuitry they are connected to.